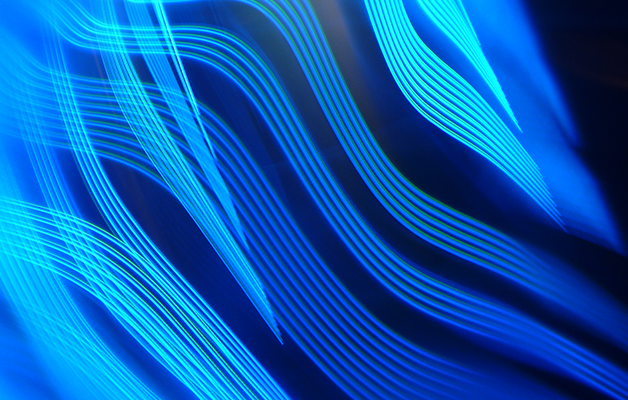
Precision Noise Measurements and Low-noise Signal Generation
Precision noise measurements and generation of low-phase noise microwave signals are two “sides of the same coin.” Recent progress in those fields was primarily associated with microwave circuit interferometry - a powerful noise measurement technique resulting from our quest to detect Gravitational Waves (GW) in the mid-90s.
The point is that the principles of the high-resolution measurements of tiny mechanical forces in the GW experiments can be applied to detect weak phase and amplitude fluctuations of microwave signals. This realization resulted in the development of microwave oscillators with significantly reduced phase noise [1-4]. Since the first applications of microwave circuit interferometry, the method continuously evolved, resulting in noise measurements with spectral resolution beyond the thermal noise limit [5, 6] and allowing the characterization of noise properties of the microwaves synthesized from the stable optical sources [7-9].
Interferometric noise measurement system
Legend
DUT – Device under test
LNA – Low-noise amplifier
FFT – Fast Fourier Transform analyzer
Microwave signal transmitted through the Device Under Test (DUT) interferes destructively with a fraction of the incident signal at the interferometer' dark port'. As a result of such interference, the carrier of the 'dark port' signal is suppressed, while the noise modulation sidebands caused by non-thermal fluctuations in the test sample remain unaffected. These modulation sidebands are amplified and converted into the voltage noise by a readout system consisting of the Low Noise Amplifier (LNA), mixer, and a phase-shifter. Depending on the setting of the phase shifter, the output voltage noise varies synchronously, either with phase or amplitude fluctuations of the DUT.
The resolution of the interferometric measurements approaches the standard thermal noise limit. The main reason is the LNA's low effective noise temperature, which is about 50K for LNAs based on high-electron-mobility transistors. Besides, one can increase the measurement resolution by simply increasing the power of the input signal, as long as the interferometer remains balanced. Finally, the interferometric measurements are first-order insensitive to the pump oscillator phase & intensity fluctuations, as long as the DUT is non-dispersive and linear. In such a case, technical fluctuations of the pump source are suppressed by the same amount as the carrier signal.
Schematic diagram of low-phase noise oscillator and its phase noise spectra at 9 GHz
Microwave sapphire-loaded cavity resonator
The figure shows a frequency-stabilized microwave loop oscillator. Here a microwave cavity-resonator serves as a band-pass filter of a self-sustaining loop oscillator and a dispersive element of a frequency discriminator.
Signal with the suppressed carrier is demodulated to DC, producing an error voltage proportional to the oscillator frequency offset from the cavity resonance. The error voltage after amplification and filtering is applied to the Voltage-controlled Phase-shifter (VCP) in the loop oscillator steering its frequency to the cavity resonance.
The frequency discriminator, filter, and VCP form a frequency control loop, which cancels oscillator frequency deviations from the resonant frequency. For the control loop of sufficiently high gain, the quality of oscillator frequency stabilization is entirely determined by the noise properties of the frequency discriminator. In this respect, an interferometric frequency discriminator shown is vastly superior to its conventional counterpart due to its lower effective noise temperature and ability to handle high signal power levels.
Experimental setup for measurements of oscillator phase fluctuations
Prototype of cryogenic microwave oscillator
Journal publications
Patents:
1. “Phase Noise Detector”, Australian Patent no. 695262, published 18.12.1995
2. “Phase Detector Using Carrier Suppression and Oscillator Using the Phase Detector”,
Patent USA no. 5 841 322, November 1998
3. Interferometric signal processing apparatus', Australian Patent no. P00242 21, May 1996
4. Phase Noise Detector, European Patent EP 0760954B1 (23.07.2003)
5. Interferometric Apparatus for producing an output signal characteristic of phase and /or amplitude noise of a device”, US patent 7505141 B2, 17.03.2009